It is the absolute limit of speed and the key to understanding the Universe. But how did scientists manage to understand how fast light can travel?
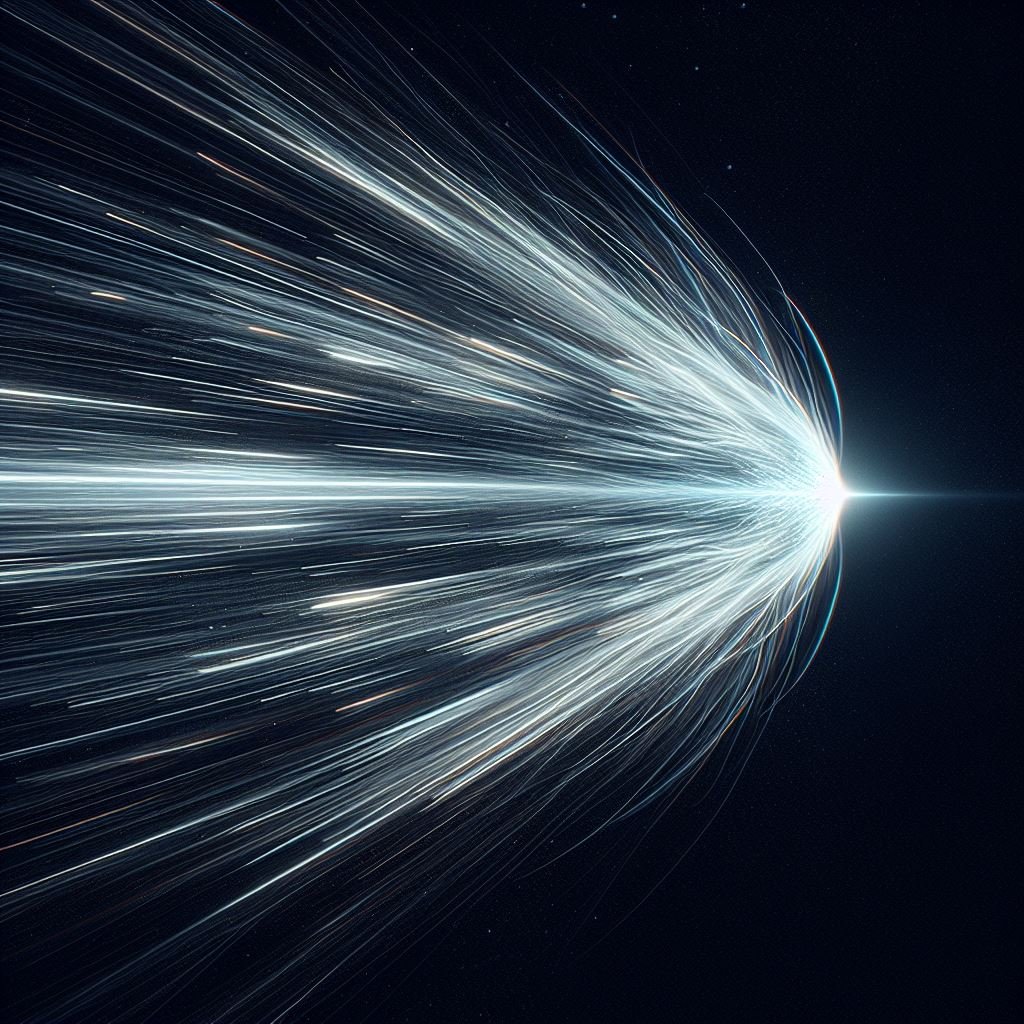
Ancient Greek mathematician Euclid believed that vision was possible thanks to rays emitted by the eyes. Hero of Alexandria claimed that light must travel with infinite speed because distant stars appear as soon as we open our eyes. And in the 11th century, the mathematician from Basra, Abu Ali al-Hasan ibn al-Haytham, wrote the “Book of Optics,” which is comparable in significance to Newton’s “Mathematical Principles of Natural Philosophy.” There, he proved that light moves from the object to the eye with a finite speed that varies depending on the properties of the medium. For example, it moves slower through glass and water than through air.
In the 13th century, Roger Bacon used al-Haytham’s ideas to argue the idea that light travels faster than sound, with a very high but finite speed. At that time, many also allowed for the possibility that light flies infinitely fast in empty space but slows down in a medium. Even in the 17th century, such luminaries of science as Kepler and Descartes claimed that light spreads infinitely fast. Kepler proved that empty space offers no resistance to its movement. Rene Descartes based his arguments on observation: during a lunar eclipse, the Sun, Earth, and Moon would noticeably deviate from a straight line if light spread at a finite speed. The absence of deviation convinced him that light spreads instantaneously. In the same era, the first attempt to directly measure the speed of light was made. In 1629, Dutch philosopher Isaac Beeckman proposed an experiment in which a flash from a cannon shot would be reflected by a mirror located about a mile away, and the difference in time between the shot and the arrival of the reflected light would be measured.
Galileo Galilei proposed an experiment with a lit lantern from which a cover was flicked off. His students tested this idea in practice in 1667. No time delay was found, so the opinion prevailed that light spreads infinitely fast. With our current knowledge of the speed of light, we understand that it took about one hundred thousandth of a second for a beam to travel there and back. This is less than the reaction time of an observer, so no one could estimate the delay—the distance in the experiment was simply too small. On the contrary, the distances between planets are so vast that it takes light several minutes to travel from one planet to another. All you need is some reference point on which to base the measurement of time intervals between events. Giovanni Cassini in Paris observed Jupiter’s moons, which, moving along their orbits, disappeared behind the planet and then reappeared. His measurements gave different results, and he explained this by the fact that light has a finite speed. Danish astronomer Ole Rømer, who worked as an assistant to Cassini from 1672 to 1681, presented the results of his observations at a meeting of the French Academy of Sciences in 1676. It turned out that Io, Jupiter’s largest moon, took less time to appear from behind the planet when Earth approached Jupiter than when it moved away from it. This confirmed Cassini’s hypothesis: when Earth approaches Jupiter, it covers part of the distance while light is on its way, and the total distance that light must travel is less. As a result, it arrives at the place relatively earlier. Conversely, when we move away, light flies further and arrives relatively later. Rømer’s measurements and the correlation he found between the results of these measurements and the Earth’s movement became the basis for recognizing him as the discoverer.
In 1690, Dutch mathematician Christiaan Huygens used Rømer’s data and obtained a value for the speed of light of 220,000 km/s, which is about 70% of the modern value. The next chapter in this story is also related to astronomy, namely to the aberration of light — a phenomenon paradoxically familiar to anyone who has walked in the rain. Suppose the rain falls vertically when you stand still. Walk forward, and it will seem that the drops fall from a point in front of you — we tilt the umbrella to stay dry. Turn it in the opposite direction, and it will seem that the source of raindrops will move in the opposite direction. Imagine that the rain is light coming from a distant star, and your movement is the movement of the Earth through space. The aberration of light leads to a change in the apparent position of the star as a result of the Earth’s movement. This phenomenon was discovered in 1729 by the future director of the Greenwich Observatory, Royal Astronomer James Bradley. He measured the position of a star in the constellation Draco and found that its position in the sky moved first to the south and then to the north over six months. The displacement was less than 1/100 of a degree, but it was easily detected with 18th-century instruments. Based on this, Bradley established that light moves 10.2 thousand times faster than the Earth in its orbit, at a speed of 295 thousand km/s. This estimate is only 2% less than.
DOWN TO EARTH
To measure high speeds, one needs to either deal with large distances, as in astronomy, or work with very small time intervals. French physicist Louis Fizeau in 1849 found a way to do this on Earth.
He passed light between the teeth of a rapidly rotating wheel. A mirror 8 km away from him reflected the light back. If the beam hit the gap between the teeth, it was visible; otherwise, darkness was observed. By changing the speed of the rotating wheel, one could determine how much time light took to travel there and back. Knowing the distance to the mirror, Fizeau was able to establish the speed of light—about 313,000 km/s. In 1862, Leon Foucault used a similar idea, using rotating mirrors to determine the angle at which light was reflected. Foucault found that the speed of light was 299,796 km/s, which is very close to the modern value—299,792.46 km/s.
In 1864, James Clerk Maxwell published a paper on electromagnetic waves. He regarded light as transverse oscillations of electric and magnetic fields propagating in space, interacting with each other. In a vacuum, electromagnetic radiation propagates without attenuation over any distance, undergoing periodic changes in intensity and induction. Induction and field intensity in a vacuum are related to the electric and magnetic constants. Previously, the terms used were (respectively) dielectric and magnetic permeability of vacuum.
In a sense, Kepler was right centuries ago. If space offers no resistance (in Maxwell’s theory, if electric or magnetic “viscosity” is zero), the speed of light will indeed be infinite. But in reality, electric and magnetic resistances are not zero, and when their values were substituted into Maxwell’s equations at the end of the century, they yielded the number 299,788 km/s—the most accurate available value of the speed of light at the time.
In 1887, Albert Michelson and Edward Morley in the USA attempted to measure the speed of the Earth relative to the luminiferous ether—a hypothetical medium believed at the time to permeate all space—by measuring the difference in the speed of light propagation in two perpendicular directions. They used semi-transparent mirrors, which reflected part of the light at 90°, while the rest passed through them without changing direction. When two beams were reflected back along the same path and combined, a difference in the speed of motion in different directions along the luminiferous ether would lead to a phase shift of two waves—non-coincidence of peaks and troughs. This would result in an interference pattern—dark and light stripes. Michelson and Morley’s setup, to their surprise, demonstrated that the speed of light is universal and independent of direction. This, in turn, led Albert Einstein to deny the existence of the ether (at least in the form it was then conceived) and to the emergence of the special theory of relativity in 1905. Thus, precise measurements of the speed of light laid the foundation for profound insights into the nature of space and time.
In particular, Einstein’s theory implies that the speed of light in a vacuum is the limit of speed in nature: there is no object with mass that can reach it. At the same time, any massless particles must travel in a vacuum at this universal speed.
But light slows down as it passes through a transparent medium, such as water or glass. Such particles as electrons can move through the medium faster than light but slower than light in a vacuum. Before the invention of the laser, independent measurements of the frequency and wavelength of electromagnetic waves were made in the 1950s using “volume resonators,” yielding a value of 299,792 km/s with an error of 3 km/s.
A modern version of this experiment can be conducted by placing a chocolate bar in a microwave oven. Remove the rotating turntable—the experimental sample should remain in place and melt faster in those points where the waves are most intense. The distances between two consecutive spots are half the wavelength of the microwave radiation used in the microwave. Multiply the wavelength by the frequency of microwave radiation (usually 2.45 GHz, but check the microwave oven manual), and you get the speed of light, albeit with less accuracy than in the laboratory in the 1950s. Modern experiments over long distances involve sending radio signals to various spacecraft. Thus, the speed of light can be calculated to twenty trillionths.
Modern descendants of Michelson–Morley technology use laser beams, the frequency of which is known with high accuracy. After the beam is split into two and then recombined, the interference pattern can be used to determine the wavelength of light. Speed is the product of wavelength and frequency. In 1972, this method allowed the precision of speed measurement to be increased to values better than four billionths.
Today, advanced high-stability lasers and measurements of time intervals using atomic clocks have provided the most accurate values—299,792.458 km/s, with an uncertainty of only 1 m/s. The duration of a second can be determined very accurately using atomic clocks, and the uncertainty in measuring the speed of light mainly depends on the accuracy of defining the meter. Subsequently, since 1983, an agreement has been reached to fix the value of the speed of light at the above value and to define the meter as 1/299,792,458 of the distance that light travels in a vacuum in one second. Today, we define the meter through the measured speed of light.
Five key terms describing the essence of light:
- Light aberration: An optical phenomenon where stars appear to shift from their true positions in the sky. This is a result of the finite speed of light and the movement of the Earth.
- Atomic clocks: The most accurate method of time measurement currently available. It utilizes the frequency of microwave signals emitted by electrons transitioning between energy levels.
- Volume resonator: A hollow conductor closed at both ends where an electromagnetic wave can be sustained by reflecting off its walls. By adjusting the parameters of the resonator and the wavelengths, running or standing waves can be produced.
- Dielectric permittivity: Electric charge generates an electric field. The property of an insulating (dielectric) medium that impedes the formation of this field is dielectric permittivity. The relative dielectric permittivity of a substance is determined by comparing the capacitances of a test capacitor with a dielectric and the same capacitor in a vacuum.
- Magnetic permeability: The measure of how easily a medium is filled with a magnetic field. The speed of light in a vacuum is inversely proportional to the square root of the product of the dielectric permittivity of the vacuum and its magnetic permeability.